|
Clean-Energy
Engineering |
|
|
|
Concentrated
solar photovoltaic systems are potentially cost-effective as they
reduce the number of solar cells required to generate a given amount
of electricity. This is done by focusing more solar radiation onto
a given cell area, with concentration ranging from tens to thousands
of effective suns. Solar radiation that is not directly converted to
electricity remains as thermal energy, and excess heat increases
cell temperature, which decreases solar-to-electricity conversion
efficiency. Therefore, effective cooling is imperative for solar
cells under high concentration. We developed a two-phase cooling
strategy for concentrated solar photovoltaic systems [1,2], and our analysis
indicated that the practical limits of solar concentration
to be about 2000 suns for the six organic fluids examined, and 4000
and 6000 suns for water and ammonia, respectively.
[1] T. Ho, S. S. Mao, and R. Greif,
“Improving efficiency of high-concentrator photovoltaics by cooling
with two-phase forced convection.” International Journal of
Energy Research, V.34, 1257 (2010).
[2]
T. Ho, S. S. Mao, and R. Greif, “The
impact of cooling on cell temperature and the practical solar
concentration limits for photovoltaics.” International Journal of
Energy Research, V.35, 1250
(2011).
|
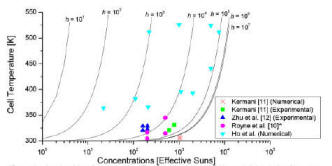
|
|
|
There
is a considerable interest in the design of functional
materials and devices for solar energy conversion, in anticipation
of the renewable energy needs associated with limited
resource of fossil fuels [1]. Of a range of materials that have been
investigated in pursuit of greater utilization of solar energy,
titanium dioxide is one of the most effective semiconductor photocatalysts, which has an electronic band gap in the
ultraviolet wavelength regime. Narrowing the band gap is
therefore vital to achieve efficient absorption of sunlight. While
doping is a well-established method of tuning the band gap, its
application to titanium dioxide has only limited
success. We demonstrated [2] a conceptually different approach to
enhancing solar absorption by tailoring disorders in nanophase
titanium dioxide through hydrogenation. We showed that
disorder-engineered titanium dioxide nanocrystals
exhibited substantial solar-driven photocatalytic activity and are
capable of producing hydrogen from water at room temperature.
[1]
X. Chen,
S. Shen, L. Guo, and S. S. Mao, “Semiconductor-based photocatalytic
hydrogen generation.” Chemical Reviews,
V.110, 6503 (2010).
[2] X. Chen, L. Liu, P. Y. Yu, and
S. S. Mao, “Increasing Solar Absorption for Photocatalysis with
Black, Hydrogenated Titanium Dioxide Nanocrystals.” Science,
V.331, 746
(2011). |
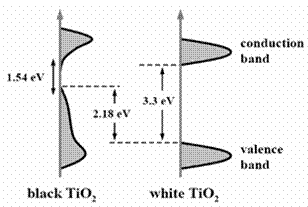
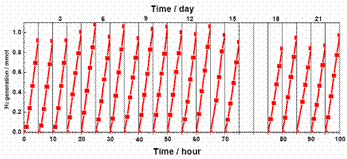
|
|
|
Organic light-emitting diodes (OLEDs) are attracting much attention
due to their potential for solid-state lighting and flat-panel
display applications. Although much effort has been devoted to the
optimization of OLED performance, carrier injection barriers between
the organic materials and the electrodes remain a limiting
factor. For both polymer and small molecule based OLEDs, we
demonstrated [1] that a carbon nanotube (CNT) layer can act as an electron injection
enhancer at the cathode-organic interface. Along a different path,
to achieve
better spectral range and photo-stability, we developed [2] a
proof-of-concept quantum dot-OLED hybrid device and
demonstrated efficient energy transfer from the blue-emitting polymer to the
red-emitting quantum dots.
[1] D. Liu, M. Fina, J. Guo, X. Chen, G. Liu, S.
Johnson, and S. S. Mao, “Organic
light-emitting diodes with carbon nanotube cathode-organic layer.”
Applied Physics Letters,
V.94, 013110 (2009).
[2] Z.-S. Guo, L. Zhao, J. Pei, Z.-L. Zhou, G.
Gibson, J. Brug, S. Lam, and S. S. Mao, “CdSe/ZnS nanoparticle
composites with amine-functionalized polyfluorene derivatives for
polymeric light-emitting diodes.”
Macromolecules,
V.43,
1860 (2010).
|
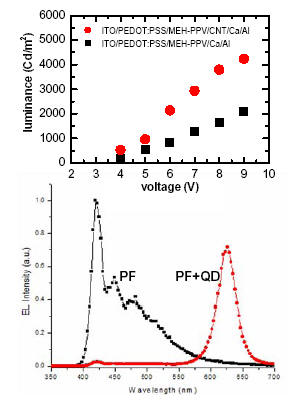
|
|
|
Hydrogen storage as a high pressure gas is energy and volume
intensive, while storage in the liquid form requires extremely low
temperatures. So solid-state hydrogen storage is attractive from the
technological point of view, but has encountered tremendous
challenges in terms of practical storage capacity and kinetics. MgH2
has been a model metal hydride for studying the fundamental
processes related to solid-state hydrogen storage. Due to its high
enthalpy of formation, bulk MgH2 generally does not
release hydrogen below 300
oC, so there is a need to
improve the thermodynamics of MgH2 such as reducing the
enthalpy of formation. We developed [1] a free-standing nanoparticle
film approach using Pd/Mg/Pd structure to improve
hydrogen uptake and release kinetics of Mg nanoparticles as well as
reduce enthalpy of formation. In addition, we are examining
oxide porous materials with large surface area (over 1000 m2/g)
and open porosity (more than 90% porous) for their potential as
hydrogen storage media [2].
[1] S. Barceló, M. Rogers, C. P.
Grigoropoulos, and S. S. Mao, “Hydrogen storage property of
sandwiched magnesium hydride nanoparticle thin film.”
International Journal of Hydrogen Energy,
V.35, 7232 (2010).
[2] S. S. Mao and X. Chen, “Selected nanotechnologies
for renewable energy applications.”
International
Journal of Energy Research,
V.31, 619 (2007).
|
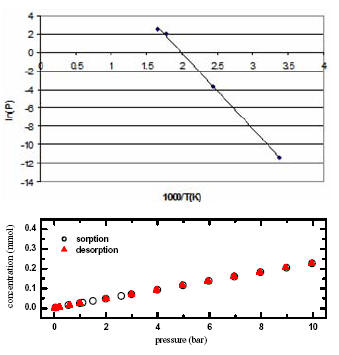
|
|
|
As clean energy conversion devices, polymer electrolyte membrane
fuel cells (PEMFCs) have many advantages, including high energy
conversion efficiency, which make them attractive for both mobile
and stationary applications. While platinum (Pt) has been regarded
as the most active catalyst for oxygen reduction reaction, the
ultimate applications of PEMFCs are hindered, at least partially,
due to the high cost of the noble material. We recently demonstrated
[1] that highly crystalline CrN nanoparticles of fcc structure exhibit
attractive catalytic activity and stability for the oxygen reduction
reaction in PEMFCs. The result offers a possible solution to the
need of cost-effective electrocatalyst alternatives for fuel cells.
Additionally, we are also developing solid-oxide fuel cells (SOFCs)
with
perovskite-phase BaSrCoFeO (BSCF) thin film cathodes prepared on
NiO-YSZ/YSZ composite substrates using pulsed laser deposition (PLD)
and traditional screen-printing method [2]. The electrochemical
performance of the SOFC cathode fabricated by PLD outperformed that
based on the screen-printing method, which can be attributed to the
smaller internal resistance within the cathode and the interfacial
resistance between the cathode and the electrolyte. This work
demonstrated the potential of PLD as a promising cathode deposition
technique for achieving high performance SOFC.
[1] H.
Zhong, X. Chen, H. Zhang, M. Wang, and S. S. Mao, “Proton exchange
membrane fuel cells with chromium nitride nanocrystal catalysts.”
Applied Physics Letters,
V.91,
163103 (2007).
[2] B. Liu, X. Chen,
Y. Dong, S. S. Mao, and M. Cheng, “A high performance
nanostructured BaSrCoFeO cathode for solid-oxide fuel cells.”
Advanced Energy Materials,
V.1,
343 (2011). |
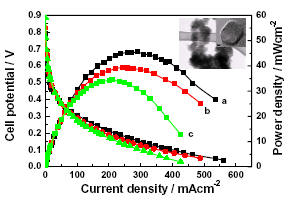
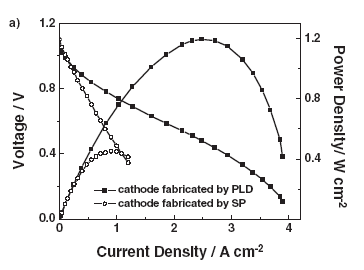
|
|
|
There is a need of thermal management to control battery temperature
as the trend to high power densities for vehicles would result in
more heat generated within a smaller volume. A temperature increase
of 15
oC
could reduce the life of a Li-ion cell by 50%. Typically Li-ion
cells have two electrodes separated by a thin membrane separator.
When charging, electrons are conducted through the external
electrical connection, and Li+ ions diffuse through the separator
towards the negative electrode. When discharging, electrons and Li+
ions flow in the opposite direction. We studied through numerical
modeling the effects of material and design modifications on the
temperature distribution of Li-ion cells, such as the incorporation
of an internal structure for better heat transfer, and the use of a
carbon nanotube negative electrode and of separators made of Separion and
different ceramics mixed with functioning particles.
[1] M. Sievers, U. Sievers, and S. S. Mao, “Thermal
modeling of new Li-ion cell design modifications.”
Engineering Research / Forschung im Ingenieurwesen,
V.74, 215 (2010). |
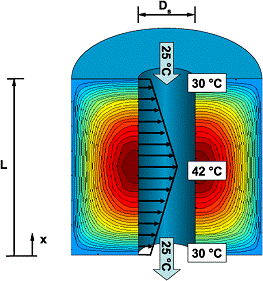 |
|
|
Since the discovery of extreme surface wetting phenomenon induced by
ultraviolet (UV) photocatalysis,
titanium dioxide has become the
material of choice for environmentally friendly applications such as
self-cleaning and anti-contamination coatings. Nevertheless, it
remains a significant challenge to realize surfaces exhibiting
persistent super-hydrophilicity but without the need of external
stimuli, especially UV irradiation that also degrades the wetting
surface over a period of time. We demonstrated [1] a porous
titanium dioxide
nanostructure that shows super-hydrophilicity without the need of
light activation, and with stability against successive wetting-dewetting
cycles. The wetting surface exhibits high optical transmittance over
a wavelength range from near UV to the infrared, enabling practical
anti-fogging technologies where transparency is critical.
[1] V. Zorba, X. Chen, and S. S. Mao,
“Superhydrophilic TiO2 surface without photocatalytic
activation.” Applied Physics Letters,
V.96,
093702 (2010). |
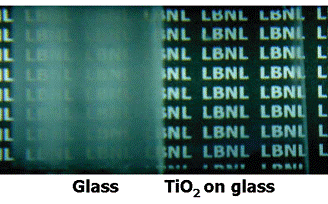 |
|
|
Clean-Energy
Engineering
Enabling Technologies
|
|
University of California at Berkeley |
this page is
under construction |
|
|